Aurora Borealis -
Northern Lights
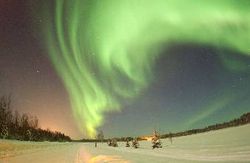
The aurora is a glow observed in the night
sky, usually in the polar zone. For this reason some scientists call it a
"polar aurora" (or "aurora polaris"). In northern latitudes, it is known
as "aurora borealis" which is Latin for "northern dawn" since in Europe
especially, it often appears as a reddish glow on the northern horizon as
if the sun were rising from an unusual direction. The aurora borealis is
also called the "northern lights". The aurora borealis most often occurs
from September to October and March to April. Its southern counterpart,
"Aurora Australis", has similar properties.
The aurora is now known to be caused by
electrons of typical energy of 1-15 keV, i.e. the energy obtained by the
electrons passing through a voltage difference of 1,000-15,000 volts. The
light is produced when they collide with atoms of the upper atmosphere,
typically at altitudes of 80-150 km. It tends to be dominated by emissions
of atomic oxygen--the greenish line at 557.7 nm and (especially with
electrons of lower energy and higher altitude) the dark-red line at 630.0
nm. Both these represent "forbidden" transitions of atomic oxygen from
energy levels which (in absence of collisions) persist for a long time,
accounting for the slow brightening and fading (0.5-1 sec) of auroral
rays. Many other lines can also be observed, especially those of molecular
nitrogen, and these vary much faster, revealing the true dynamic nature of
the aurora.
Auroras can also be observed in the ultra-violet (UV) light, a very good
way of observing it from space (but not from ground--the atmosphere
absorbs UV). The "Polar" spacecraft even observed it in X-rays. The image
is very rough, but precipitation of high-energy electrons can be
identified.
Typically the aurora appears either as a
diffuse glow or as "curtains" that approximately extend in the east-west
direction. At some times, they form "quiet arcs", at others ("active
aurora") they evolve and change constantly. Each curtain consists of many
parallel rays, each lined up with the local direction of the magnetic
field lines, suggesting that aurora is shaped by the Earth's magnetic
field, Indeed, satellites show auroral electrons to be guided by magnetic
field lines, spiralling around them while moving earthwards.
The curtains often show folds called "striations". When the field line
guiding a bright auroral patch leads to a point directly above the
observer, the aurora may appear as a "corona" of diverging rays, an effect
of perspective.
In 1741 Hiorter and Celsius first noticed other evidence for magnetic
control, namely, large magnetic fluctuations occurred whenever the aurora
was observed overhead. This indicates (it was later realized) that large
electric currents were associated with the aurora, flowing in the region
where auroral light originated. Kristian Birkeland (1903) deduced that the
currents flowed in the east-west directions along the auroral arc, and
such currents, flowing from the dayside towards (approximately) midnight
were later named "auroral electrojets"
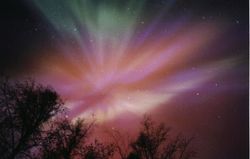
a corona
Still more evidence for a magnetic connection
are the statistics of auroral observations. Elias Loomis (1860) and later
in more detail Hermann Fritz (1881) established that the aurora appeared
mainly in the "auroral zone", a ring-shaped region of approx. radius 2500
km around the magnetic pole of the Earth, not its geographic one. It was
hardly ever seen near that pole itself. The instantaneous distribution of
auroras ("auroral oval", Yasha Feldstein 1963) is slightly different,
centred about 3-5 degrees nightward of the magnetic pole, so that auroral
arcs reach furthest equator-ward around midnight.
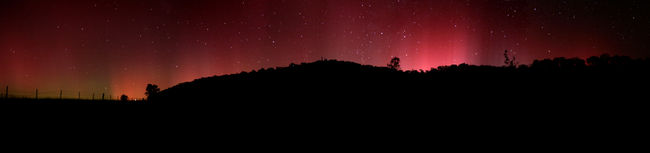
Aurora Australis
the solar wind and magnetosphere
The Earth is constantly immersed in the solar
wind, a rarefied flow of hot plasma (gas of free electrons and positive
ions) emitted by the sun in all directions, a result of the million-degree
heat of the sun's outermost layer, the solar corona. The solar wind
usually reaches Earth with a velocity around 400 km/s, density around 5
ions/cc and magnetic field intensity around 2–5 nT (nanoteslas; the
Earth's surface field is typically 30,000–50,000 nT). These are typical
values. During magnetic storms, in particular, flows can be several times
faster; the interplanetary magnetic field (IMF) may also be much stronger.
The IMF originates on the sun, related to the field of sunspots, and its
field lines (lines of force) are dragged out by the solar wind. That alone
would tend to line them up in the sun-earth direction, but the rotation of
the Sun skews them (at Earth) by about 45 degrees, so that field lines
passing Earth may actually start near the western edge ("limb") of the
visible sun.
The Earth's magnetosphere is the space region dominated by its magnetic
field. It forms an obstacle in the path of the solar wind, causing it to
be diverted around it, at a distance of about 70,000 km (before it reaches
that boundary, typically 12,000–15,000 km upstream, a bow shock forms).
The width of the magnetospheric obstacle, abreast of Earth is typically
190,000 km, and on the night side a long "magnetotail" of stretched field
lines extends to great distances.
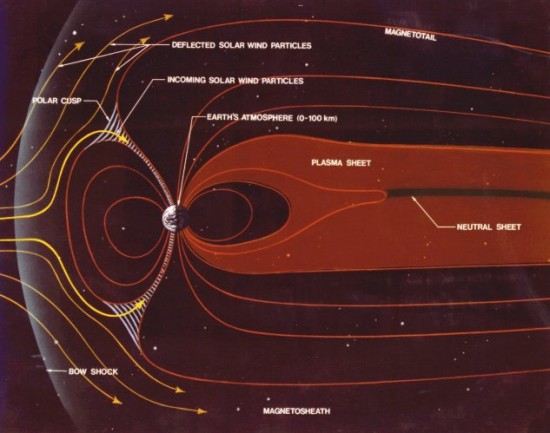
frequency of occurrence
The aurora is a common occurrence in the
ring-shaped zone. It is occasionally seen in temperate latitudes, when a
strong magnetic storm temporarily expands the auroral oval. Large magnetic
storms are most common during the peak of the 11-year sunspot cycle, or
during the 3 years after that peak. However, within the auroral zone the
likelihood of an aurora occurring depends mostly on the slant of IMF lines
(known as Bz, pronounced "bee-sub-zed"), being greater with southward
slants.
Geomagnetic storms that ignite auroras actually happen more often during
the months around the equinoxes. It is not well understood why geomagnetic
storms are tied to the Earth's seasons when polar activity is not. It is
known, however, that during spring and autumn, the earth's and the
interplanetary magnetic field link up. At the magnetopause, Earth's
magnetic field points north. When Bz becomes large and negative (i.e., the
IMF tilts south) it can partially cancel Earth's magnetic field at the
point of contact. South-pointing Bz's open a door through which energy
from the solar wind can reach Earth's inner magnetosphere.
The peaking of Bz during this time is a result of geometry. The
interplanetary magnetic field comes from the Sun and is carried outward
the solar wind. Because the Sun rotates the IMF has a spiral shape.
Earth's magnetic dipole axis is most closely aligned with the Parker
spiral in April and October. As a result, southward (and northward)
excursions of Bz are greatest then.
However, Bz is not the only influence on
geomagnetic activity. The Sun's rotation axis is tilted 7 degrees with
respect to the plane of Earth's orbit. Because the solar wind blows more
rapidly from the Sun's poles than from its equator, the average speed of
particles buffeting Earth's magnetosphere waxes and wanes every six
months. The solar wind speed is greatest -- by about 50 km/s, on average
-- around Sept. 5th and March 5th when Earth lies at its highest
heliographic latitude.
Still, neither Bz nor the solar wind can fully explain the seasonal
behaviour of geomagnetic storms. Those factors together contribute only
about one-third of the observed semi-annual variation.
the origin of the aurora
The ultimate energy source of the aurora is
undoubtedly the solar wind flowing past the Earth.
Both the magnetosphere and the solar wind consist of plasma, which can
conduct electricity. It is well known (since Faraday's work around 1830)
that if two electric conductors are immersed in a magnetic field and one
moves relative to the other, while a closed electric circuit exists which
threads both conductors, then an electric current will (usually) arise in
that circuit. Electric generators of dynamos make use of this process
("the dynamo effect"), but the conductors can also be plasmas or other
fluids.
In particular the solar wind and the magnetosphere are two electrically
conducting fluids with such relative motion, and should be able (in
principle) to generate electric currents by "dynamo action", in the
process also extracting energy from the flow of the solar wind. The
process is hampered by the fact plasmas conduct easily along magnetic
field lines but not so perpendicular to them. It is therefore important
that a temporary magnetic interconnection be established between the field
lines of the solar wind and those of the magnetosphere, by a process known
as magnetic reconnection. It happens most easily with a southward slant of
interplanetary field lines, because then field lines north of Earth
approximately match the direction of field lines near the north magnetic
pole (namely, into the earth), and similarly near the southern pole.
Indeed, active auroras (and related "substorms") are much more likely at
such times.
Electric currents originating in such fashion apparently give auroral
electrons their energy. The magnetospheric plasma has an abundance of
electrons: some are magnetically trapped, some reside in the magnetotail,
and some exists in the upwards extension of the ionosphere, which may
extend (with diminishing density) some 25,000 km around the Earth.
Bright auroras are generally associated with
Birkeland currents (Schield et al., 1969; Zmuda and Armstrong, 1973) which
flow down into the ionosphere on one side of the pole and out on the
other. In between, some of the current connects directly through the
ionospheric E layer (125 km), the rest ("region 2") detours, leaving again
through field lines closer to the equator and closing through the "partial
ring current" carried by magnetically trapped plasma. The ionosphere is an
ohmic conductor, so such currents require a driving voltage, which some
dynamo mechanism can supply. Electric field probes in orbit above the
polar cap suggest voltages of the order of 40,000 volts, rising up to more
than 200,000 volts during intense magnetic storms.
Ionospheric resistance has a complex nature, and leads to a secondary Hall
current flow. By a strange twist of physics, the magnetic disturbance on
the ground due to the main current almost cancels out, so most of the
observed effect of auroras is due to a secondary current, the auroral
electrojet. An auroral electrojet index (measured in nanotesla) is
regularly derived from ground data, and serves as a general measure of
auroral activity.
However, ohmic resistance is not the only obstacle to current flow in this
circuit. The convergence of magnetic field lines near Earth creates a
"mirror effect" which turns back most of the down-flowing electrons (where
currents flow upwards), inhibiting current-carrying capacity. To overcome
this, part of the available voltage appears along the field line
("parallel to the field"), helping electrons overcome that obstacle by
widening the bundle of trajectories reaching Earth; a similar "parallel
voltage" is used in "tandem mirror" plasma containment devices. A feature
of such voltage is that it is concentrated near Earth (potential
proportional to field intensity; Persson, 1963) and indeed, as deduced by
Evans (1974) and confirmed by satellites, most auroral acceleration occurs
below 10,000 km. Another indicator of parallel electric fields along field
lines are beams of upwards flowing O+ ions observed on auroral field
lines.
While this mechanism is probably the main source of the familiar auroral
arcs, formations conspicuous from the ground, more energy might go to
other, less prominent types of aurora, e.g. the diffuse aurora (below) and
the low-energy electrons precipitated in magnetic storms.
Some O+ ions ("conics") also seem accelerated in different ways by plasma
processes associated with the aurora. These ions are accelerated by plasma
waves, in directions mainly perpendicular to the field lines. They
therefore start at their own "mirror points" and can travel only upwards.
As they do so, the "mirror effect" transforms their directions of motion,
from perpendicular to the line to lying on a cone around it, which
gradually narrows down.)
In addition, the aurora and associated currents produce a strong radio
emission around 150 kHz known as auroral kilometric radiation (AKR,
discovered in 1972). Ionospheric absorption makes AKR observable from
space only.
These "parallel voltages" accelerate electrons to auroral energies and
seem to be a major source of aurora. Other mechanisms have also been
proposed, in particular, Alfvén waves, wave modes involving the magnetic
field first noted by Hannes Alfvén (1942), which have been observed in the
lab and in space. The question is however whether this might just be a
different way of looking at the above process, because this approach does
not point out a different energy source, and many plasma bulk phenomena
can also be described in terms of Alfvén waves.
Other processes are also involved in the aurora, and much remains to be
learned. Auroral electrons created by large geomagnetic storms often seem
to have energies below 1 keV, and are stopped higher up, near 200 km. Such
low energies excite mainly the red line of oxygen, so that often such
auroras are red. On the other hand, positive ions also reach the
ionosphere at such time, with energies of 20-30 keV, suggesting they might
be an "overflow" along magnetic field lines of the copious "ring current"
ions accelerated at such times, by processes different from the ones
described above.
|